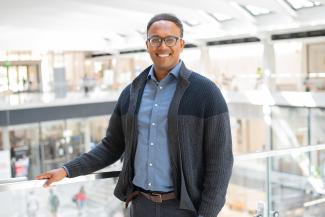
Shwetadwip Chowdhury, assistant professor in the Chandra Family Department of Electrical and Computer Engineering at The University of Texas at Austin, has received a National Institutes of Health grant for $1.8 million over five years.
This award will support Chowdhury’s research project to develop a major technological breakthrough that could revolutionize our ability to study how organisms develop. By developing novel computational optics imaging technologies, Chowdhury’s research team aims to capture at unprecedented scales 3D views of how tissues and organs form, a process known as morphogenesis. This new approach could provide critical insights into how organisms grow from a single cell to complex structures made of trillions of cells.
Optical imaging has long been a crucial tool for studying biological processes like morphogenesis because it allows researchers to visualize live samples with incredible detail. Unlike other methods, such as X-rays or MRIs, optical imaging can capture non-invasive, real-time images of biological tissue at the cellular to subcellular level. However, optical imaging faces a significant challenge: light scatters as it passes through biological tissue, scrambling vital information and limiting the depth at which we can see clearly. Current state-of-the-art optical imaging technologies for deep-tissue imaging, like confocal and multiphoton microscopes, can only penetrate about one millimeter into tissue before light scattering obscures the image. This poses a major hurdle for studying morphogenesis in developing organisms, which undergo growth across multiple scales throughout their developmental cycle.
Interestingly, though scattered light appears chaotic and random, it actually encodes tissue-specific information across centimeter-scale distances. If scattered light could be fully unscrambled, an order-of-magnitude deeper imaging would be possible. Technologies like adaptive optics aim to exploit this by using wavefront-shaping elements to physically correct for scattering. However, these elements often lack the complexity needed to fully correct for tissue-scattering, limiting scatter correction to small fields of view insufficient for visualizing tissue morphogenesis at 3D whole-organism scales.
To overcome this, Chowdhury’s research team is developing new optical imaging technologies that computationally correct for scattering. Computational correction of scattering (e.g., inverse-scattering) is limited mainly by the practical constraints on computing power, which is constantly improving. If successful, this strategy could allow the team to 3D visualize heterogeneously scattering tissues on whole-organism scales and at longer depths than ever before. This approach combines sophisticated computer models with cutting-edge optical hardware design to reconstruct high-resolution, large field-of-view, and extended-depth images in challenging conditions where light scattering would normally be a barrier.
Chowdhury’s research team has already achieved promising preliminary results suggesting this approach is feasible. For instance, Siqi Yang, a 4th-year Ph.D. student on the team, recently developed an innovative coded-illumination optical system that can project specific patterns onto 3D biological samples, encoding sample-specific scattering information into the raw measurements. By feeding these measurements into a computational inverse-scattering reconstruction algorithm developed in the lab, scattering effects can be corrected, enabling 3D reconstruction of the sample’s morphological structure. This technique has been successfully applied to visualize early-stage zebrafish embryos.
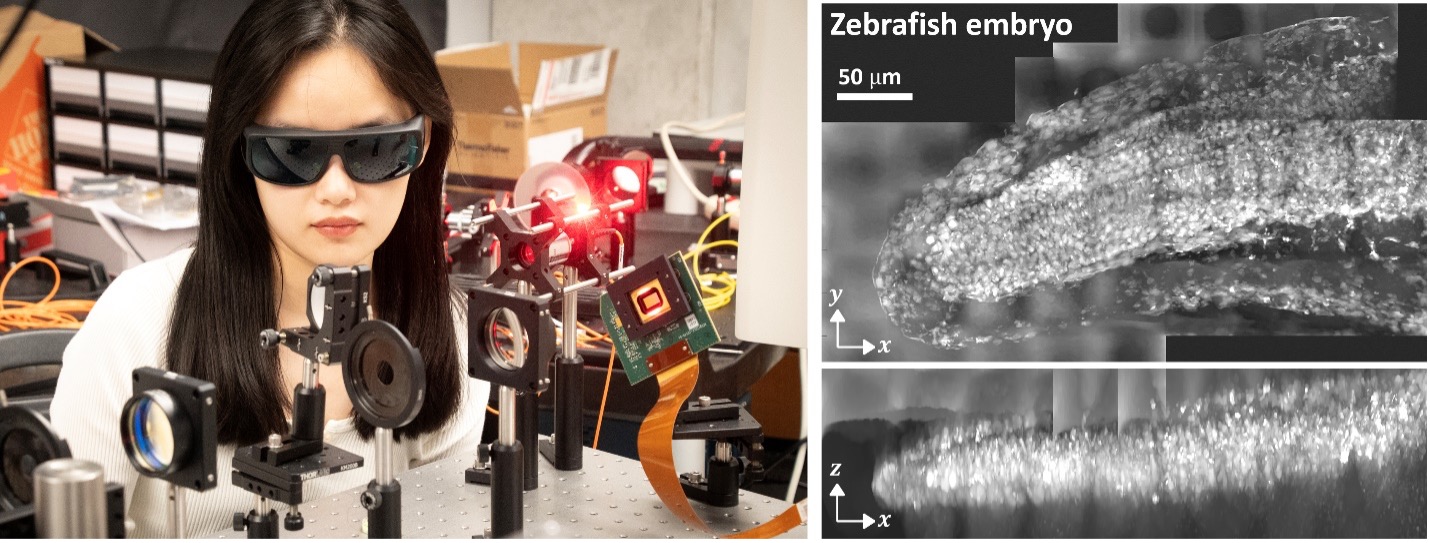
Siqi Yang aligns a coded-illumination optical system to collect scattered light from embryo samples. Computational reconstruction enables label-free visualization of the sample’s morphological features, as recently demonstrated with early-stage zebrafish embryos
Building on this initial success, Chowdhury’s research team is now pushing the limits of this method. Jeongsoo Kim, a 3rd-year Ph.D. student on the team, is imaging calibrated scattering samples of various sizes and scattering strengths, 3D printed with sub-micron scale features. By reconstructing the 3D structural profiles of these samples from scattering measurements and comparing them to the known printed distributions, Kim is devising inverse-scattering strategies that optimize reconstruction accuracy across a wide range of samples with varying scattering properties. These insights have significantly improved 3D reconstruction fidelity for various biologically scattering samples, such as C. elegans, organoids, and histopathology tissue sections.
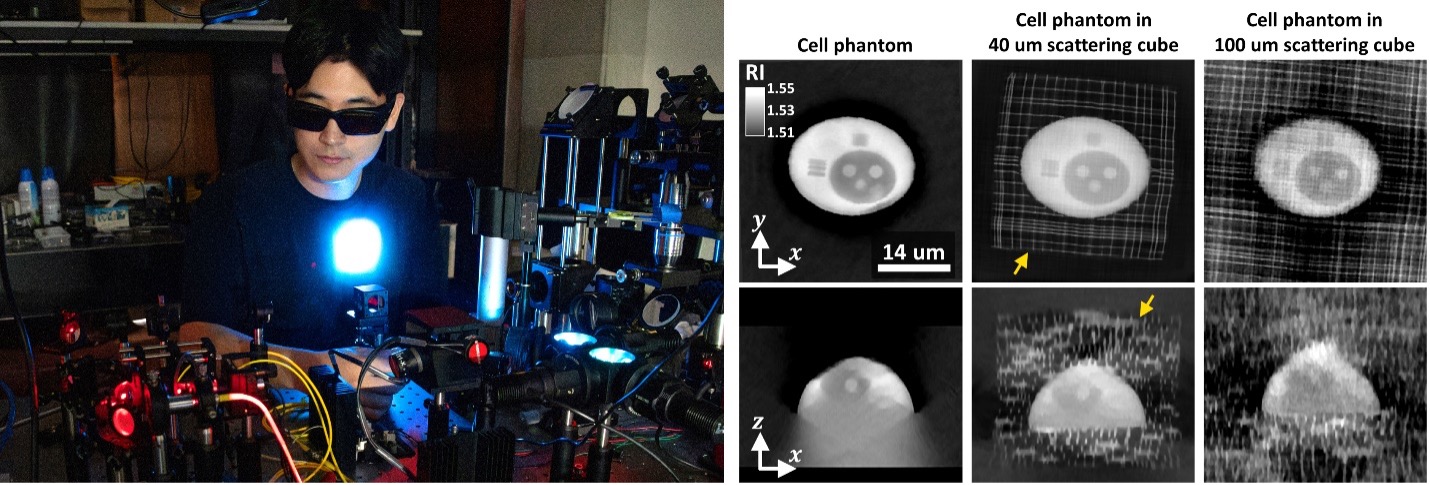
Jeongsoo Kim studies how the lab’s inverse-scattering strategies performs on various samples with different scattering properties. For example, 3D phantoms of varying sizes were used to evaluate how imaging quality depends on scattering strength. This research offers insights into factors that limit imaging performance and provides strategies to optimize image fidelity across different sample types.
Moving forward, Chowdhury’s research team will focus on applying this computational imaging method to the challenging task of imaging tissue morphogenesis. This work promises to open new doors for scientists and could one day lead to basic-science and medical research by providing a deeper understanding of how tissues develop, regenerate, and respond to disease.
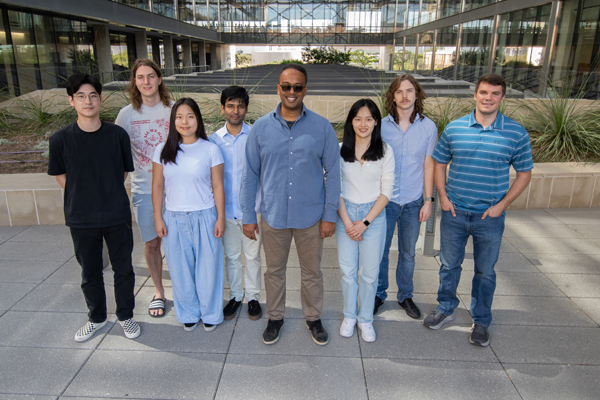
Lab photo. From left to right: Jeongsoo Kim, Peter Wagenaar, Hyobin Cho, Sibi Chakravarthy Shanmugavel, Prof. Shwetadwip Chowdhury, Siqi Yang, Dakota Britton (co-advised student), Grant Brown (undergraduate)